Hydrogen is at our disposal, so it seems, in many colours: black, grey, green, blue, turquoise, purple, aquamarine, pink, red, blue, yellow, orange, white, … [1, 2, 7]. Some colours are relatively new, like pink or purple for hydrogen from nuclear power stations, and white or orange for hydrogen originating from some rock formations. But the story about the colours is mixed up with fairy tales and advertisement. A division on the basis of real differences may make hydrogen production more understandable than all those colours. In reality, hydrogen is colourless. And the chosen colours indicate rather than describe which is misleading. We can produce hydrogen from water, methane (natural gas) or both. ‘Flash hydrogen’ from natural gas may be the best option. But that has not yet come through to policy makers and the public at large.
Since the unrestricted release of carbon dioxide (CO2) in the atmosphere has been recognized as the main driver of present climate change, researchers have looked for alternatives to the present hydrogen production technologies. Alternatives that do not release CO2 to the atmosphere.
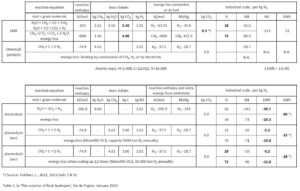
SMR, hydrogen from water and natural gas
The conventional process, used from the end of the 19th century onwards, leads steam through coal to produce ‘water gas’ (town gas) [3]. This was done in a closed reactor vessel, heated by coal combustion. This ‘coal gasification’ produced hydrogen (H2) and carbon monoxide (CO). This mixture was also being used as ‘syngas’ in chemical industry, for subsequent synthesis of ‘organic’ materials and plastics. Since the rise of the oil industry, methane (CH4) is the common resource for this process instead of coal (C).
In a subsequent step, the ‘water gas shift reaction’, the carbon monoxide will react further with steam to carbon dioxide and more hydrogen [table 1]. In sum, this process produces hydrogen (H2) and carbon dioxide (CO2) from steam (H2O) and methane (CH4); it is therefore called Steam Methane Reforming (SMR, also called MSR). The hydrogen produced partly originates from water, partly from natural gas.
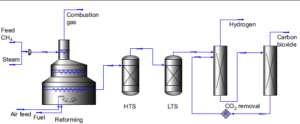
In the SMR process, part of the natural gas is being used to heat the reactor vessels. This heat will eventually dissipate to the environment, being lost. SMR is being used since 1930 and has been largely perfected. In is a complicated process with a number of reactions and purifications [figure 1]. Through the use of catalysts, reaction temperature could be lowered from 1000oC to 800oC. This implies less use of natural gas and less wear of the reactor vessels.
On average, the SMR process will produce 9.5 kg of CO2 per kg of hydrogen [5]. World-wide, also coal, lignite or fossil oil are still being used for hydrogen production; and therefore, the global average for all fossil fuels results in 12 kg CO2 per kg of hydrogen.
Stop the discharge of CO2 to the atmosphere
There are two ways to keep CO2 out of the air during the production of hydrogen. Either we choose a process for hydrogen production without CO2 as a byproduct, or we try to store safely the CO2 that results from the process, for instance in empty natural gas fields. The latter is called CCS: Carbon dioxide Capture and Storage. In short, this is the choice between prevention and cure.
As an alternative to SMR, petrochemical industry chose SMR plus CCS. And they also came up with a colour: blue. Billions are being invested in SMR-hydrogen plus CCS (blue hydrogen), among which major tax subsidies. Nevertheless, the process will only capture 70% of all CO2 produced; the remainder still ends up in the atmosphere. We cannot afford to capture more than 70% CO2, and we cannot arrive at 100% [6]. We can only capture CO2 where we burn a lot of natural gas in one location. CCS is not a solution for all use of natural gas as a fuel. Large-scale CO2 storage has not yet been realized anywhere.
A second look at hydrogen production technologies
We need a clearer difference between hydrogen production technologies than on the basis of colour. What are the resources used? What production processes can we perform, and what are the differences? What is the hydrogen yield of each process? What happens with any CO2 produced? People who will have to decide on the future of all those hydrogen colours, like Dutch parliamentarians in 2021, do not seem to have a clue on the exact nature of methane splitting processes, apart from single exceptions.
Unknown, unloved. The choice would seem to be between electrolysis, what the ‘greens’ want; or store CO2 in empty gas fields, what the ‘greys’ from the petrochemical industries prefer. Other options still have to be discovered, so it seems.
But concepts like ‘enthalpy’, ‘plasma’ and ‘pyrolysis’ aren’t common knowledge. Not with parliamentarians, nor with the media or the average citizen. In those circumstances, it will be difficult to judge the differences between SMR+CCS, pyrolysis, electrolysis and plasmolysis. And even more difficult, if the question is how much hydrogen can be made in each process, using a certain amount of electricity. The Dutch Wikipedia doesn’t know the concept of ‘methane pyrolysis’, and checking the lemma ‘pyrolysis’ isn’t very helpful either. But then, looking for ‘grey hydrogen’, one finds a large number of colours. Although the English version is more informative.
Hydrogen production resources and splitting technologies
Two resources are in ample supply for the production of hydrogen: natural gas (CH4) and water (H2O). Each of these resources can be used on its own, or combined like in SMR. Recovering hydrogen from water requires 7.6 times a much energy as recovery from natural gas [table 1].
Each of these reactions requires energy – not just the energy required to produce hydrogen, but also energy for the maintenance of the required conditions, like temperature. The latter energy will eventually end up in the environment, as heat loss.
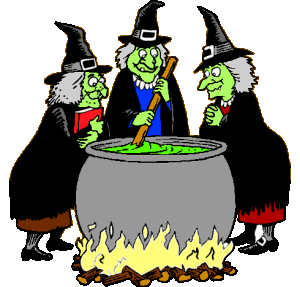
The way in which the various processes are supplied with the required energy leads to an essential distinction. With both SMR and ‘pyrolysis’, reactor vessels are supplied with extra energy from the outside, usually by burning natural gas or hydrogen. That is ‘normal’, traditional chemistry, the art of splitting chemical substances. Just as you can ‘stoke’ a brew in a pot with a fire [Figure2]. Only that pot is now called a ‘reactor vessel’, a special pot that is closed off from the environment. And the process is called ‘pyrolysis’, ‘splitting with fire’. If the temperature is high enough, almost everything decomposes.
Modern ways to split substances use electricity instead of fuel: electrochemistry and plasma chemistry. In both cases, electricity is introduced directly into the reaction mixture, providing the energy for the conversion and maintaining the reaction mixture at the correct reaction conditions.
Anyone who has ever charged a car battery knows that hydrogen can be released. If you pass direct current through water in which a salt has been dissolved, hydrogen bubbles up at one of the electrodes. This is a different way of making hydrogen than the traditional one, via electrolysis of water. No energy is required to heat the battery from outside. The electricity does its separating and heating work inside the battery. The electrical resistance requires extra energy.
We can do even more with electricity. We can use it to generate electrical discharges with high voltages, as happens naturally in lightning. Under those conditions, matter decomposes into electrons, ions and radicals. One then speaks of a ‘plasma’. Plasma is a fourth ‘aggregation state’, in addition to solid, liquid and gas. Plasmas can be generated in different ways. We are already completely familiar with the ‘cold’ plasmas in fluorescent tubes and TV screens.
What happens in a plasma reactor for the splitting of natural gas into hydrogen gas and carbon powder is most similar to lightning [See Fig. 2]. A continuous ‘arc’ is maintained between the electrodes. Gases that are guided through such an electric arc decompose into the elements. Under the right reaction conditions, 100% of methane splits into hydrogen and carbon.
Unfortunately, the term ‘methane pyrolysis’ obscures the difference between ‘pyrolysis’ and ‘plasma lysis’ (or ‘plasmolysis’) of methane. It also does not become clearer that hydrogen made through these very different processes is referred to with one color: ‘turquoise’. And the use of the distinguishing terms ‘thermal pyrolysis’ and ‘plasma pyrolysis’ is also not illuminating.
Perhaps the distinction between ‘traditional hydrogen’, ‘battery hydrogen’ and ‘lightning hydrogen’ (or flash hydrogen) is more appealing.
The actual state of affairs
Official bodies and journalists suggest that methane pyrolysis is still in its infancy, and that SMR+CCS is a mature, large-scale technology. That is a fairy tale, that’s in the interest of the oil majors with their existing SMR equipment, empty gas fields and craving for subsidies. It just isn’t true. Yes, at the Dutch research centre TNO researchers investigate ‘conventional methane pyrolysis’, but nowhere in the Netherlands ‘flash hydrogen’ is made. And no, CO2 storage is still in its infancy.
Table 1 compares SMR and the three alternative hydrogen production technologies that do not produce any CO2: pyrolysis, electrolysis and plasmolysis. Pyrolysis runs into the same problems that partly haunt SMR: finding a good catalyst that doesn’t deactivate fast, and reaction products separation [9]. ‘Thermal pyrolysis’ of methane isn’t available at an industrial scale yet.
CO2-free alternatives to SMR
Applicable on an industrial scale at present there are just two CO2-free alternatives to SMR: electrolysis and plasmolysis. At present, plasmolysis at Monolith requires 25 kWh/kg of hydrogen. Electrolysis on the average requires 60 kWh/kg of hydrogen. Which means that plasma pyrolysis needs less than half of the electricity required for electrolysis. After scaling up at Monolith, this could come down to less than one third: 18 kWh/kg of hydrogen [9]. Under those circumstances, plasmolysis will even beat SMR without CCS, from an energy point of view.
At present, the Netherlands exclusively focuses on SMR+CCS and electrolysis of water. There was a plan to build an electrolysis plant in Delfzijl, but this has been shelved. It would have a capacity of 3000 to 9000 tons of hydrogen annually. Now, there is an application for an 800 MW electrolysis factory in the Rijnmond area. It should produce up to 80 000 tons of hydrogen annually: the Eneco Electrolyser. Investment in this plant is supposed to be € 100 million.
A plasmolysis factory on the other hand would produce 3 times as much hydrogen, using the same amount of electricity from wind turbines in the North Sea. This would satisfy directly the goal of 3x as much in 2030 [12]. Or, looked at this from the other side, the Eneco Electrolyser would waste 70% of the green electricity fed into it. Electricity that might have been put to a better use by feeding it into the grid, allowing a faster reduction of fossil energy in the electricity production.
The choice for electrolysis instead of plasmolysis is a missed chance. Moreover, this project will stand in the way of a faster energy transition. Would there be a scientific detective in the Netherlands who could investigate this, and discuss it with specialists? Like with the Dutch ‘hydrogen’ professor emeritus Ad van Wijk and with French professor Laurent Fulcheri, head of research at Mines ParisTech and promotor of the American plasmolysis company Monolith in Nebraska.
TNO’s René Peters, who promotes blue hydrogen production in the Rotterdam harbour, is silent about pyrolysis or plasmolysis. One of his arguments against electrolysis technology is that it cannot be scaled up quickly enough (because of a shortage of electrolysers) in order to be significant for total hydrogen production in the Rijnmond area. And that therefore blue hydrogen is the only option for a fast energy transition. But how fast can natural gas plasmolysis be scaled up? Just ask Robert Hanson, founder and CEO of Monolith Corporation.
The future of methane splitting
Plasmolysis of natural gas will produce very pure carbon powder as a side product (because natural gas is not contaminated with solids or liquids). That powder can be used for soil improvement and for industrial purposes, like ‘carbon black’ in motor car tyres, toners for laser printers and new materials based on carbon. If too much side product is being produced, this can simply be stored, unlike CO2, that requires an elaborate and costly infrastructure.
An objection against the continued use of natural gas for hydrogen production is that in the entire production chain, from well to end product, gas leaks occur. In Europe that amounts to a few parts per thousand. But in the USA, where natural gas is being extracted by ‘fracking’ of claystone, leakages may amount to as much as 3.8% [4]. That natural gas is being transported to Europe by LNG tankers. In this process, another 20% of the natural gas recovered is being used for compressing, cooling and transport.
If one should use this imported natural gas for methane splitting, the hydrogen produced will not be 100% CO2-free anymore. The same applies to electrolysis of water with electricity from the grid. One could however prevent such leaking losses by locating the methane splitting installations near to the natural gas wells.
Natural gas from wells at the Norwegian shore plays an important role in Europe. Even though the use of Dutch natural gas is largely tabooed at the moment, the Netherlands do have a large infrastructure for importing, use and transit of gases to and from the North Sea. And an electricity network to and from wind turbine parks. North Sea natural gas could possibly be converted into hydrogen, using wind park electricity. That can be done through electrolysis of water or through plasmolysis of methane. The latter option looks better, as the electricity price is the main factor in the business case.
On a global scale, the use of CO2-free methane splitting technologies might have far-reaching implications for the energy transition [8]. We can expect methane plasmolysis to make a breakthrough in countries with major natural gas reserves and lots of sunlight, like Qatar. As soon as it turns out that the choice for electrolysis has disadvantages in terms of efficiency and returns.
Conclusion
The plasmolysis process is superior to all other processes considered.
– Steam Methane Reforming (SMR) is an undesirable process, as it will release much CO2. And because we have not yet mastered the art of storing all CO2 in the underground (if we ever will).
– Hydrogen production through electrolysis requires (too) much energy.
– Plasmolysis is a proven technology, in the Monolith factory in Nebraska (USA). Its total energy use can even be lowered by scaling up the process. It is the most energy efficient process available. It coproduces very pure carbon, that can either be used or simply stored.
Interesting? Then also read:
Energy forecasts
Strict environmental regulation will stimulate sustainable innovation
RMI: decarbonize fast and profitably
References
[1] Nationalgrid (2023). The hydrogen colour spectrum
[2] IDTechEx (2023). The spectrum of hydrogen colors
[3] Snijders, A.J.C. (1885). Het watergas, de brandstof der toekomst
[4] Howarth, R.W. (2015). Methane emissions and climatic warming risk from hydraulic fracturing and shale gas development: implications for policy. Energy and Emission Control Technologies, 2015(3), 45-54
Alvarez, R.A., et.al.(2018). Assessment of methane emissions from the U.S. oil and gas supply chain. Science, 361(6398), 186-188
Foulds, A., et.al. (2022). Quantification and assessment of methane emissions from offshore oil and gas facilities on the Norwegian continental shelf. Atmospheric Chemistry and Physics, 22, 4303-4322
Pühl, M., et.al. (2024). Aircraft-based mass balance estimate of methane emissions from offshore gas
facilities in the southern North Sea. Atmospheric Chemistry and Physics, 24, 1005-1024
[5] Fulcheri, L. (2021). Methane pyrolysis: The third way for low CO2 hydrogen production, 18-03-2021, fsr.eui.eu/between-green-and-blue-a-debate-on-turquoise-hydrogen
[6] Brandl, P., et.al. (2021). Beyond 90% capture: Possible, but at what cost? International Journal of Greenhouse Gas Control, 105, 103239
[7] Ajanovic,A., et.al. (2022) The economics and the environmental benignity of different colors of hydrogen. International Journal of Hydrogen Energy, 47(57), 24136-24154
[8] Diab, J., et.al. (2023). Why Turquoise Hydrogen Will Be a Game Changer for The Energy Transition. International Journal of Hydrogen Energy, 47(61), 25831-25848
[9] Fulcheri, L., et.al. (2023). An energy-efficient plasma methane pyrolysis process for high yields of carbon black and hydrogen. International Journal of Hydrogen Energy, 48(8), 2920-2928
[10] Lee, Y.H., et.al. (2023). Evaluation of process conditions for methane pyrolysis applying the triple thermal plasma system. International Journal of Hydrogen Energy, 48(70), 27127-27136
[11] Wnukowski, M. (2023). Methane Pyrolysis with the Use of Plasma: Review of Plasma Reactors and Process Products. Energies, 16, 6441-6474
[12] Port of Rotterdam (2023). Planned 800-MW Eneco Electrolyser brings the target of 2.5 GW hydrogen production in 2030 closer
Also check: climatedata.nl